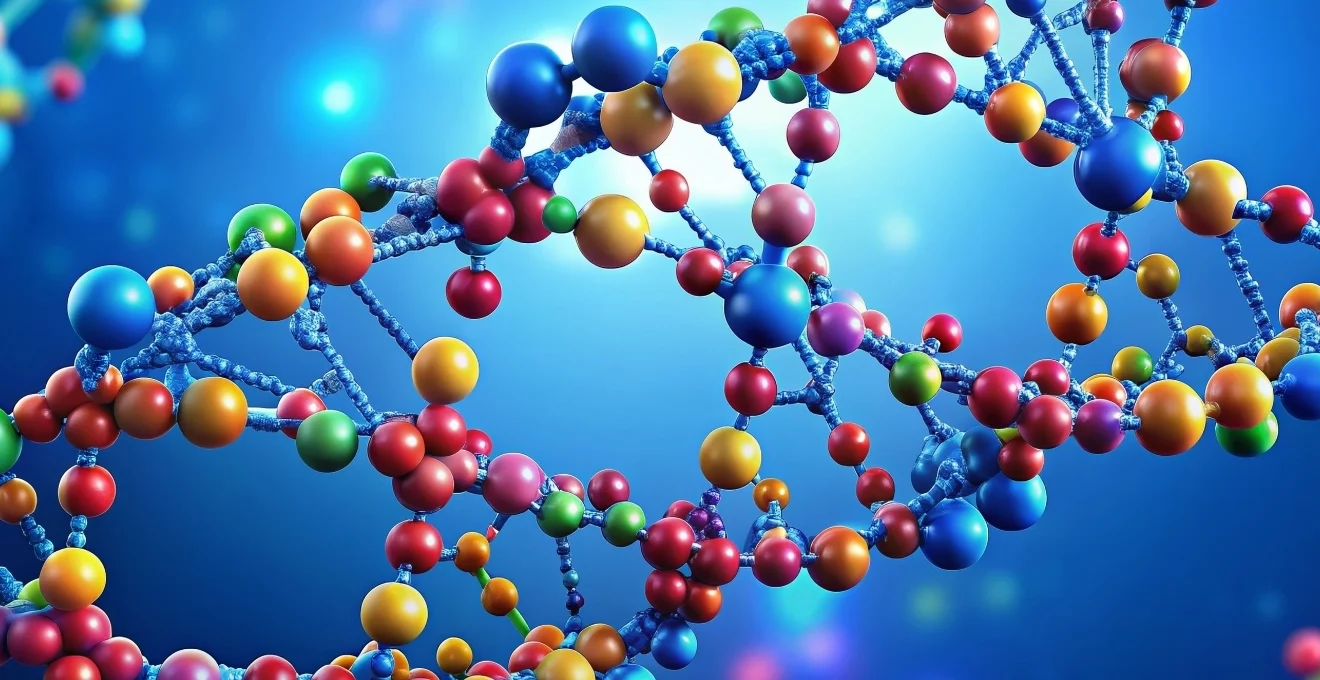
Aptamers and antibodies represent two powerful classes of molecular recognition tools that have revolutionized diagnostics, therapeutics, and research applications. These biomolecules exhibit remarkable abilities to bind specific targets with high affinity and selectivity. However, their distinct molecular structures, production methods, and binding mechanisms lead to important differences in their affinity and specificity profiles.
Molecular structure and binding mechanisms of aptamers and antibodies
Aptamers are short, single-stranded oligonucleotides (DNA or RNA) that fold into unique three-dimensional structures capable of binding specific targets. Their structural flexibility allows them to form intricate shapes like hairpins, pseudoknots, and G-quadruplexes. This conformational adaptability enables aptamers to recognize a wide range of targets, from small molecules to large proteins.
In contrast, antibodies are large, Y-shaped proteins composed of two heavy chains and two light chains. The antigen-binding site, located at the tips of the Y, is formed by the variable regions of these chains. This protein structure provides a stable scaffold for target recognition but offers less conformational flexibility compared to aptamers.
The binding mechanisms of aptamers and antibodies differ significantly. Aptamers typically interact with their targets through a combination of hydrogen bonding, van der Waals forces, and shape complementarity. Their ability to undergo conformational changes upon binding allows for induced fit interactions, potentially leading to high-affinity complexes.
Antibodies, on the other hand, primarily rely on the spatial arrangement of amino acids in their complementarity-determining regions (CDRs) to form specific interactions with antigens. The rigid protein structure of antibodies often results in a lock-and-key type of binding mechanism, which can be highly specific but may limit the range of potential targets.
Affinity comparison: aptamers vs antibodies
Affinity, a measure of the strength of binding between a ligand and its target, is a critical parameter in evaluating the performance of molecular recognition tools. Both aptamers and antibodies can exhibit high affinities for their respective targets, but there are notable differences in the range and distribution of affinities observed for these two classes of molecules.
Dissociation constants (kd) in Aptamer-Target complexes
Aptamers typically demonstrate dissociation constants (Kd) ranging from the picomolar to low micromolar range. The flexibility of aptamer structures allows for the selection of molecules with exceptionally high affinities. For example, some aptamers targeting proteins have been reported with Kd values in the low picomolar range, rivaling or even surpassing the affinities of the best monoclonal antibodies.
One of the advantages of aptamer technology is the ability to fine-tune affinity through iterative selection processes. By adjusting selection conditions, researchers can optimize aptamers for specific affinity ranges suitable for various applications. This flexibility is particularly valuable in developing diagnostic tools where precise control over binding strength is crucial.
Antibody-antigen binding kinetics
Antibodies generally exhibit dissociation constants in the nanomolar to low micromolar range. High-affinity monoclonal antibodies can achieve Kd values in the sub-nanomolar range, making them excellent tools for applications requiring strong and stable binding. The protein-based structure of antibodies provides a stable scaffold for antigen recognition, often resulting in slower dissociation rates compared to aptamers.
It's worth noting that while some aptamers can match or exceed antibody affinities, the distribution of affinities for antibodies tends to be more consistent across different targets. This consistency is partly due to the natural evolution of the immune system to produce high-affinity antibodies against a wide range of antigens.
Impact of environmental factors on binding affinity
The binding affinities of both aptamers and antibodies can be significantly influenced by environmental factors such as pH, temperature, and ionic strength. However, aptamers often show greater sensitivity to these conditions due to their nucleic acid nature. This sensitivity can be both an advantage and a challenge, depending on the application.
For instance, aptamers can be designed to exhibit dramatic changes in affinity in response to specific environmental triggers, making them valuable for developing smart biosensors or stimuli-responsive drug delivery systems. Antibodies, with their more stable protein structure, generally maintain their binding properties across a broader range of conditions, which can be advantageous in certain in vivo applications.
The environmental sensitivity of aptamers allows for the development of sophisticated molecular switches, while the stability of antibodies ensures consistent performance across diverse physiological conditions.
Specificity analysis: aptamer selection vs antibody production
The specificity of molecular recognition tools is crucial for their effectiveness in various applications, from diagnostics to targeted therapeutics. Aptamers and antibodies achieve their specificity through fundamentally different processes, each with its own advantages and limitations.
SELEX process for aptamer development
Aptamers are developed through a process called Systematic Evolution of Ligands by EXponential enrichment (SELEX). This in vitro selection method allows for precise control over the conditions under which aptamers are selected, enabling the generation of highly specific binders. The SELEX process typically involves the following steps:
- Incubation of a large random oligonucleotide library with the target molecule
- Separation of bound sequences from unbound ones
- Amplification of bound sequences
- Repetition of the process with increasing stringency
One of the key advantages of SELEX is the ability to perform negative selection steps, where sequences that bind to undesired targets are removed from the pool. This feature allows for the development of aptamers with exquisite specificity, capable of distinguishing between closely related molecules or even different conformational states of the same protein.
Monoclonal antibody generation via hybridoma technology
Monoclonal antibodies are typically produced using hybridoma technology, which involves the following steps:
- Immunization of an animal (usually a mouse) with the target antigen
- Isolation of B cells producing antibodies against the target
- Fusion of B cells with myeloma cells to create immortal hybridomas
- Screening and selection of hybridomas producing desired antibodies
This in vivo process relies on the natural immune system to generate specific antibodies. While it can produce highly specific antibodies, it is limited by the immune tolerance of the host animal, making it challenging to generate antibodies against certain conserved proteins or small molecules.
Cross-reactivity profiles of aptamers and antibodies
Cross-reactivity, the ability of a molecular recognition tool to bind unintended targets, is a critical consideration in many applications. Aptamers and antibodies exhibit different cross-reactivity profiles due to their distinct selection and production processes.
Aptamers, through careful design of the selection process, can achieve extremely low cross-reactivity, even against structurally similar molecules. The ability to perform iterative negative selections allows for the fine-tuning of specificity. For example, aptamers have been developed that can distinguish between enantiomers or phosphorylated and non-phosphorylated versions of a protein.
Antibodies, while often highly specific, can sometimes exhibit cross-reactivity with structurally similar antigens. This is particularly true for polyclonal antibodies, which consist of a mixture of antibodies recognizing different epitopes on the same antigen. Monoclonal antibodies generally offer improved specificity but may still show some level of cross-reactivity, especially when targeting conserved protein domains.
It's important to note that while aptamers can theoretically be selected against any target, including toxic or non-immunogenic molecules, antibodies are limited to targets that can elicit an immune response in the host animal. This difference expands the range of potential applications for aptamers in certain fields, such as environmental monitoring or detection of small molecule contaminants.
For a more detailed comparison of the advantages of aptamers over antibodies, you can refer to https://novaptech.com/en/aptamers-vs-antibodies-advantages/
Applications leveraging differential affinities and specificities
The unique affinity and specificity profiles of aptamers and antibodies have led to their adoption in a wide range of applications, each leveraging the strengths of these molecular recognition tools.
Aptamer-based biosensors and diagnostics
Aptamers have found particular success in the development of biosensors and diagnostic tools. Their small size, ease of chemical modification, and ability to undergo conformational changes upon target binding make them ideal for creating sensitive and specific detection systems. Some notable applications include:
- Electrochemical sensors for rapid detection of pathogens
- Fluorescence-based assays for small molecule detection
- Lateral flow devices for point-of-care diagnostics
The ability to select aptamers with specific binding kinetics allows for the development of sensors with rapid response times or controlled release profiles. Additionally, the stability of DNA aptamers enables the creation of robust sensors capable of functioning in harsh environmental conditions.
Antibody-dependent cellular cytotoxicity in immunotherapy
Antibodies excel in applications that leverage their ability to interact with the immune system. One of the most significant areas where this is evident is in cancer immunotherapy, particularly through antibody-dependent cellular cytotoxicity (ADCC). In this process, antibodies bind to specific antigens on cancer cells and recruit immune effector cells to destroy the target.
The Fc region of antibodies, which is absent in aptamers, plays a crucial role in engaging immune cells and complement proteins. This unique feature of antibodies makes them particularly effective in therapeutic applications where modulation of the immune response is desired.
Comparative performance in protein purification techniques
Both aptamers and antibodies are used in affinity chromatography for protein purification, but their different properties lead to distinct advantages in specific scenarios.
Aptamers offer several benefits in protein purification:
- Chemical stability allows for harsh elution conditions
- Ease of regeneration for multiple uses
- Ability to design aptamers for specific binding and elution conditions
Antibodies, on the other hand, are often preferred when:
- High binding capacity is required due to their larger size
- Purification of proteins from complex biological samples is needed
- The target protein is present at very low concentrations
The choice between aptamers and antibodies in protein purification often depends on the specific requirements of the purification process, such as scale, target protein stability, and desired purity level.
The complementary strengths of aptamers and antibodies in various applications highlight the importance of selecting the appropriate molecular recognition tool based on the specific requirements of each project.
Challenges and limitations in affinity and specificity optimization
While both aptamers and antibodies offer powerful molecular recognition capabilities, they each face unique challenges in optimizing affinity and specificity for certain applications.
For aptamers, one of the primary challenges is maintaining structural integrity and function in complex biological environments. The nuclease sensitivity of RNA aptamers can limit their effectiveness in certain in vivo applications. While chemical modifications can enhance stability, these alterations may impact binding affinity or specificity.
Antibodies face challenges related to their production process. The reliance on animal immunization can make it difficult to generate antibodies against toxic compounds or highly conserved proteins. Additionally, batch-to-batch variability in polyclonal antibody production can lead to inconsistencies in affinity and specificity profiles.
Both aptamers and antibodies can exhibit off-target effects, albeit through different mechanisms. Aptamers may interact non-specifically with other nucleic acids or nucleic acid-binding proteins, while antibodies can engage Fc receptors on cells not intended as targets. Mitigating these off-target interactions often requires careful design and extensive validation.
The optimization of affinity and specificity for both aptamers and antibodies often involves trade-offs. For instance, increasing affinity may come at the cost of reduced specificity, as tighter binding interactions may be less discriminatory. Balancing these parameters to achieve optimal performance for a given application remains an ongoing challenge in the field.
Future directions: engineering enhanced aptamers and antibodies
The field of molecular recognition is continuously evolving, with researchers exploring innovative approaches to enhance the performance of both aptamers and antibodies. Several promising directions are emerging:
For aptamers, the development of mirror-image aptamers, or spiegelmers
, offers a potential solution to the stability issues faced by traditional aptamers. These L-RNA or L-DNA molecules are highly resistant to nuclease degradation while maintaining the binding properties of their natural counterparts.
Another exciting area is the creation of aptamer-small molecule conjugates, which combine the specificity of aptamers with the pharmacological properties of small molecules. These hybrid molecules could potentially overcome some of the limitations of traditional aptamer therapeutics, such as rapid renal clearance.
In the antibody field, advances in protein engineering are leading to the development of bispecific and multispecific antibodies capable of engaging multiple targets simultaneously. These engineered antibodies hold promise for more effective cancer therapies and treatment of complex diseases.
Computational approaches are playing an increasingly important role in optimizing both aptamers and antibodies. Machine learning algorithms are being employed to predict binding affinities and specificities, potentially streamlining the selection and optimization process for both classes of molecules.
The integration of aptamers and antibodies into more complex molecular systems is another area of active research. For example, aptamer-antibody chimeras combine the best features of both molecules, offering unique possibilities for diagnostic and therapeutic applications.
As our understanding of molecular recognition deepens and new technologies emerge, the distinction between aptamers and antibodies may become less clear-cut. The future likely holds a diverse toolkit of molecular recognition agents, each optimized for specific applications and seamlessly integrating the strengths of various binding modalities.
The ongoing advancements in aptamer and antibody engineering promise to expand the capabilities of these molecular recognition tools, opening up new possibilities in diagnostics, therapeutics, and basic research. As the field continues to evolve, researchers and clinicians will have an increasingly sophisticated array of options for addressing complex biological challenges with unprecedented precision and efficacy.